Chapter One
Introductory
-- What are Toadstools?
-- The Ways of Life of Trees and Toadstools
'The mushrooms have two strange properties; the one that they yeeld so delicious a meat; the other, that they come up so hastily, as in a night, and yet they are unsown.'
-- Bacon, Silva Sylvarum, or a Naturall Historie in Ten Centuries, 1627.
It will not have escaped the notice of even casual observers that a relationship of some kind appears to exist between trees and toadstools; that, for example, at certain seasons of the year toadstools are specially abundant on the ground in or about woodlands and beneath trees (Pl. 2, 4, 5).
Many of these are conspicuous objects, large and often brightly coloured, and a little attention bestowed on their distribution shows that certain kinds are regularly associated with particular trees or types of woodlands, or show a constant preference for other situations. Thus, the white-flecked, scarlet caps of the Fly Agaric are common in pine and birch woods and frequently appear under isolated trees of Scots pine and Silver birch. Another common woodland toadstool, the cèpe of the French markets, is frequent in beech woods. This has a large cap, light to chestnut, brown in colour, carried on a rather swollen stem four to five inches in height. The under surface of the cap does not bear gills as is the case in the edible Mushroom or the Fly Agaric but is perforated by fine round pores, the openings of passages that tunnel the substance of the cap in a vertical direction. The truffle, actually a kind of underground toadstool, is formed below the surface of the soil among roots of beech and certain other trees, and smaller structures, similar in appearance to truffles, produced by other members of the same family of fungi are found in soil near the roots of pines and other coniferous trees.
Other common toadstools appear regularly, not in woodlands or among trees, but with grasses and in pastures. Of such are the Common Mushroom (Psalliota campestris), the Horse Mushroom (Psalliota arvensis), the Parasol Fungus (Lepiota procera), likewise an edible species, and Blewitts (Tricholoma personatum), a common vegetable dish in many parts of France and elsewhere.
Ability to recognize and identify the commoner toadstools is of some practical interest and importance. Some, for example, the Mushroom, Chantarelle (Cantharellus cibarius) and Truffle (Tuber spp.) are greatly esteemed for their culinary virtues; the orange-coloured toadstools with yellow milky juice of the Saffron Milk Cap or Delicious Lactarius (Lactarius deliciosus) were valued as food in the ancient world and are mentioned by Pliny; many others are edible, if of less outstanding merit; a few are injurious or even violently poisonous. Of these last, the scarlet Fly Agaric (Amanita muscaria) (Pl. 2, 3) with a nearly related species, the Death Cap or Death's Angel fungus (Amanita phalloides) (Pl. 4) with a pale greenish-yellow cap, common and abundant species about pine and birch trees and in woodlands formed by them, are two of the most notorious. The toadstools of both these species, especially those of the latter, contain virulent poisons and if cooked and eaten in the fresh condition, are among the most deadly and dangerous poisons known; the Panther Agaric, Amanita pantherina, a toadstool with a brown spotted cap, is also common in such woodlands and also poisonous. It is interesting to note that the fleshy caps of these poisonous toadstools are eaten with impunity by slugs.
These observations lead naturally to further inquiries. What are toadstools? What is their manner of life and how, if at all, does it differ from that of trees and other plants among which they grow? Is there any explanation for their distribution in nature; in particular, for the constant association of some kinds with certain species of trees or other plants?
In the first place: what are toadstools? According to the Oxford Dictionary, a toadstool is 'a fungus having a round disk-like top and a slender stalk, a mushroom'. Enlarging upon this definition, it is explained that the term, as used popularly, is often restricted to 'poisonous or inedible fungi' to distinguish these from edible 'mushrooms'. There is some uncertainty about the origin and exact meaning of the name toadstool. Variants such as tadstoles, todestoles, todestolys and others occur in medieval literature from 1398 onwards. It is believed that toad, found in middle English as tode and toode, refers to the animal, and this view finds support in its use in the form toodys hatte in the year 1440.
In Dutch, the name for a toad is pad; a toadstool is called paddenstoel; in Flemish paddenstool. Padok or Paddok is an old Scottish name for the frog, and in Scotland and the North of England toadstools are still called paddock- or puddockstools.
The implied relation of the frog or toad in toadstool is very puzzling. In Lyly's Euphues the following passage appears: 'I am of this minde with Homer, that as the Snayle that crept out of hir shell was turned eftsoones into a Toad, and thereby was forced to make a stoole to sit on, disdaining hir own house...' The Euphues, written between the years 1578 and 1580, was responsible for introducing a fashion for the use of picturesque similes drawn from natural history without necessary relation to accuracy of fact. Lyly's observations about the behaviour of Snayles need not therefore be taken very seriously. His 'Snayle' that 'crept out of hir shell' might indeed very easily have been a slug, still not uncommonly found browsing upon succulent toadstools. Spenser in his Shepheard's Calendar (1579) appears to have been convinced that toads sat upon toadstools:
'Where I was wont to seeke the honey Bee,
Working her formall rowmes in wexen frame,
The grieslie Tode-stoole growne there mought I se
And loathed Paddocks lording on the same.'
-- Spenser, The Shepheard's Calendar, 'December', 12, 67-70, 1579.
Botanically, mushrooms, toadstools and puff balls, moulds and mildews, all apparently so unlike, are the reproductive parts of different members of the same great group of plants, the Fungi. They produce the dust-like spores by means of which fungi in general reproduce and multiply, and these spore-bearing organs or sporophores with the spores produced by them exhibit a most bewildering variety of size, shape, colour, and structure. Incidentally, they provide useful material for recognizing the affinities of the different fungi concerned, and so offer a convenient basis for grouping and classifying these plants according to their true relationships.
Individual fungus spores are minute bodies of microscopic size showing great variety in colour, shape, and other details of structure; collectively, they resemble dust or powder of various colours, so light that the particles composing it are easily carried about by the lightest currents of air. The green powdery spores of the mould fungi can be brushed off any mouldy surface as a smear of greenish dust. The purplish-brown spores of the common mushroom or its relatives may be collected and observed by laying a recently expanded cap, gills downward, for a few hours on a white surface; the white spores of the Fly Agaric and other white-gilled forms can be put in evidence in a similar manner; owing to their colour, these are more conspicuous if collected on a sheet of black paper or other dark surface. The variously coloured spores of the cèpe (Boletus edulis), and related forms having pores instead of gills on the underside of the caps, can be collected in the same way, by placing the porous surface of a recently expanded cap on a suitably coloured surface.
Simple experiments of this kind not only enable us to recognize the spores of these fungi, but tell us something of the way in which they are produced. Thus, a ripe mushroom or other gill-bearing toadstool sheds its spores in a definite pattern corresponding to the lines of the gills, so indicating that the surface of the gills is the spore-producing or fertile region, the colour of which is directly affected by that of the myriads of spores produced on it. The forms with pores instead of gills shed their spores in little heaps each of which corresponds to the mouth of a pore; hence, the spores are evidently produced upon the surface that lines the hollow tubes of which these pores are the openings. It is interesting that there should be this restriction of spore production to certain parts of the spore-bearing organs, since in many of the less highly developed fungi spores are produced all over the surface of the sporophores. Because the spores are very light and readily moved by currents of air and the patterns formed by them when they fall thus easily disturbed, a glass or other cover should be placed over the cap when doing experiments of this kind.
Puff-balls, Earth-stars, the Bird's Nest fungus, and Truffles all produce their spores in vast number within closed structures, from which the spore dust eventually escapes by splitting of the leathery outer jacket or jackets and the breaking down of the internal walls of chambers within which it was produced. All these spore-bearing organs, whether individually simple and of microscopic size like those of the moulds, or large and conspicuous 'toadstools' as is the case with many of the higher fungi, are called sporophores (from the Greek, spore-bearers), sometimes, with less accuracy, fruit-bodies or fructifications. Those mentioned represent only a minute fraction of the vast group of plants known as fungi, a group including many thousands of species; even the single family to which the mushroom and other gill-bearing forms belong includes a bewildering number and variety of different species. A majority of the common forms that occur in woodlands belong to the latter group; their sporophores are toadstools in the strict dictionary sense. It is convenient for our present purpose, however, to include under this name the sporophores of fungi such as puff-balls of different kinds as well as the underground structures formed by certain of their allies, also the truffle fungi and their relatives.
Let us turn now to consider the fungus plant as a whole, apart from the more or less conspicuous organs concerned only with the production of spores and their dispersal in nature. In all but the simplest and most primitive forms, that part of the plant body in a fungus which corresponds to the roots and shoots of an ordinary green plant -- the vegetative as compared with the reproductive region -- consists of a system of finely branched tubular threads that ramify in all directions upon and below the surface of the material upon which the fungus is growing. These hollow threads or hyphae (singular hypha) collectively form the mycelium or plant body, by means of which a fungus carries on all the ordinary vital activities of nutrition and growth. At certain phases of growth, the vegetative mycelium produces special spore-bearing branches or systems of branches which may remain relatively simple and isolated as in the moulds and mildews, or become densely interwoven to form large and complicated sporophores such as mushrooms, toadstools, and the like.
The production of a firm felt-like structure from close interweaving of branched hyphae occurs also in the vegetative parts. Many of the higher fungi on occasion form long rope-like structures consisting of hyphae intertwined as strands in a rope; these are known as rhizomorphs, and their thickness will obviously depend on the size and number of the separate hyphae composing them in any individual case (Pl. 8, 9). Examination of the top few inches of the litter covering the ground in woodlands in damp weather in autumn usually reveals an abundance of such rhizomorphs appearing as a sparsely branched system of threads varying in thickness and colour. By some fungi specialized tuberous masses of hyphae are formed, giving rise to structures known as sclerotia; these serve as more or less permanent vegetative organs, resistant to drought and other unfavourable conditions and capable of producing active, growing hyphae when the environment again becomes favourable. Yet another example of a specialized structure built up of interwoven hyphae, and one that is of immediate interest as touching the main subject of our present inquiry, is the fungus mantle that clothes the lateral roots of many forest trees and other plants, usually put in evidence by the increase in thickness of these rootlets and their varied and often conspicuous colour (Pl. 10). It is only in the higher fungi that the isolated hyphae composing the mycelium become wefted to form these relatively massive structures, usually easily recognizable to the naked eye. As is the case in all the individual hyphae of these fungi, those composing the rhizomorphs or sclerotia are septate, that is, regularly divided up by cross-walls into separate chambers or compartments. In the lower fungi, the hyphae are not regularly segmented in this way but form continuous hollow tubes.
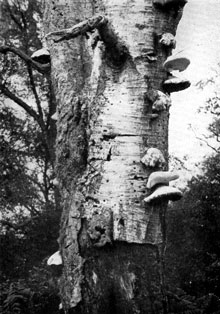
Plate 1. Sporophores of the parasitic fungus, Polyphorus betulinus, emerging from the trunk of a tree of Silver birch killed by the parasite.
|
Let us now inquire what is the way of life of a typical fungus, and how, if at all, this differs from that of a tree or other familiar green plant? As everyone knows or should know, the most important characteristic of green plants is the fact that they are green, for on this single fact -- the possession of chlorophyll -- hinges the whole mechanism of nutrition in plant and animal life as we know it in the world to-day. The bodies of plants and animals are composed of a large amount of water with a relatively small amount of solid material consisting mainly of organic substances of more or less complex chemical composition. Of the elements that form these substances, one of the most important in respect to amount is carbon, the problem of securing the supplies of this element continually required for building and repairing their bodies constituting therefore an important part of the food economy of all living organisms. The sources of carbon in nature are various. Vast supplies are locked up in the organic materials that compose the bodies of plants and animals, or in those such as sugar and starch produced by green plants; inorganic forms of carbon exist in various forms: coal, charcoal, and similar substances derived originally from organic sources; as diamonds and carbonates of various kinds present in mineral form in rocks or soil; lastly and most important of all, in the relatively small percentage but inexhaustible supply of carbon-dioxide gas in the atmosphere. This gas is present in the air in the proportion of about .03 parts in a hundred, rising to a much higher concentration in crowded rooms or other confined spaces. It is formed by the oxidation of substances containing carbon, whether rapidly as in the burning of coal, wood, or other carbonaceous materials, or slowly in the respiratory processes of animals and plants.
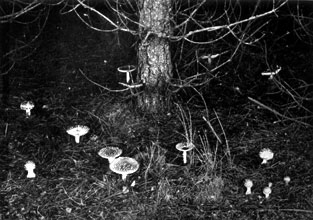
Plate 2. Sporophores of the Fly Agaric (Amanita muscaria) beneath a tree of Corsican pine (Pinus nigra) in a plantation of young trees of this species of pine. Near Wareham, Dorset.
|
Like ourselves, all other animals depend exclusively upon organic sources of carbon for their food supplies, and they must seek these in living or dead bodies of other animals or of plants, or in organic products derived from them such as starch, sugar, milk, or eggs. During and after germination young seedlings use sugar and other forms of organic carbon transferred from the reserves in the seed. It is known that mature green plants under experimental conditions can likewise absorb and utilize appreciable amounts of sugar from external sources, although it is unlikely that this method of obtaining carbon plays a part of much importance in their food economy under natural conditions. A few specialized green plants are able to satisfy part of their food requirements in the same way as do animals; for example, the so-called carnivorous species which trap insects by means of special organs, digesting the softer parts of their bodies and absorbing the soluble products of digestion in much the same way as an animal swallows, digests, and absorbs food.
So far as elemental forms of carbon are concerned, diamonds and graphite are as inaccessible to ordinary green plants as sources of carbon as they are to ourselves, as is the carbon locked up in coal or charcoal; inorganic carbon compounds such as chalk, or other soil carbonates, are equally unacceptable.
For the vast majority of green plants, the main source of carbon is the carbon-dioxide gas in the air, present in relatively small amounts, but continually renewed as an end-product of the combustion of carbon-containing or carbonaceous substances in nature, whether in course of the breathing processes of plants and animals or by other kinds of burning. By virtue of a mechanism provided by the chlorophyll in their green parts when exposed to light, green plants obtain the energy required to build up the carbon contained in carbon-dioxide into sugar and starch and other organic materials. This unique property of green plants -- the capacity to build up or synthesize the carbon contained in carbon-dioxide into organic materials and to incorporate these into their bodies, using for this purpose the energy provided by sunlight -- is known to science as photosynthesis; literally, building up by means of light.
In fungi, this remarkable food mechanism is lacking. They are without chlorophyll and, like other non-green plants and all animals, depend upon ready-made sources of carbon for their food supplies. For this reason they are of necessity parasites, growing in and upon living animals or plants and preying upon their hosts for the food they require, consuming the reserve materials stored by the latter in their bodies or (and) breaking down the organic substances of which these bodies are actually composed, or saprophytes, using the food products of green plants such as starch and sugar, or living upon organic materials derived from the decay of plant and animal bodies after death.
But carbon is only one of the essential elements that must be supplied in the foodstuffs required by all plants, green and non-green alike. The others, such as hydrogen, oxygen, nitrogen, phosphorus, etc. are obtained in the form of water and soluble mineral salts. Green plants absorb these constituents of their food by means of their roots from the soil or water in which they are growing; fungi obtain them by means of their hyphae in contact with organic materials in the soil or elsewhere, or, in the case of nitrogen and mineral salts, from the same sources as do green plants. In both cases the foodstuffs are necessarily absorbed in solution, and must therefore be present in the soil or elsewhere in forms soluble in water and otherwise suitable for absorption by roots or hyphae as the case may be, or they must be converted to such forms as a first stage in their utilization.
The raw food materials containing nitrogen are of special importance because this element is a constant and essential constituent of the proteins of which the living stuff or protoplasm of all organisms, whether plant or animal, is composed chemically, and also because the forms in which nitrogen is accessible for most green plants are rather restricted.
There is no carbon problem in the food economy of green plants, constant renewal of carbon-dioxide in the atmosphere ensuring ample supplies. There is, however, a very acute nitrogen problem, the solution of which for the ordinary green plant is closely bound up with the vital activities of various members of the soil population, among which may be reckoned the mycelium of many soil fungi.
Although roughly 75 per cent of the volume of the air we breathe consists of nitrogen in the gaseous form, this source of the element is useless for green plants, as it is indeed for all other living organisms except certain highly specialized bacteria, probably some species of fungi, and a few of the simplest green plants or algae. Next in importance in respect to amount is the nitrogen bound up chemically with carbon and other elements in the organic materials that compose the bodies of plants and animals. Of these materials, by far the greater part of the dry weight consists always of carbon and the elements composing water (H20), the proportion of nitrogen present varying from 1 per cent to 14 per cent according to the kind of plant or animal and the part of the body concerned.
As in the case of carbon, there is evidence that some green plants can absorb directly certain soluble organic substances containing nitrogen; as with carbon, however, it is unlikely that this unusual capacity plays a part of much importance in the nutrition of green plants as a whole. There exist also the small and specialized groups already noted in relation to food supplies of carbon; carnivorous plants capable of obtaining supplies of both carbon and nitrogen by trapping insects and absorbing the products of their digestion; and parasitic flowering plants that prey upon their neighbours, filching food from their shoots or roots as the case may be. Some members of the last group like the Broomrape (Orobanche spp.) and Dodder (Cuscuta spp.) have become totally dependent, lacking green leaves or the capacity to form chlorophyll; sometimes, as in the case of the Dodder, lacking also the capacity to form roots and thereby severing all direct connection with the soil.
There is also a small and equally remarkable group of flowering plants, not apparently parasitic in habit, that have lost their power of forming chlorophyll and are often regarded as having become completely saprophytic in their mode of nutrition. Whether, however, these plants, such as Indian Pipe (Monotropa Hypopitys), Bird's Nest orchid (Neottia nidus-avis), and other orchids of similar habit, are truly saprophytic and derive their food supplies directly from the fallen leaves and other organic detritus in which they commonly grow, or depend to a greater or less extent upon the activities of certain fungi invariably associated with their roots or other underground parts, is not at all certain. The latter alternative seems the more likely.
Diagram Illustrating the 'Nitrogen Cycle'
The large circle on the left represents the main 'nitrogen cycle' in which nitrates from the soil are built up into complex proteins within the plant, these being subsequently again broken down to nitrates by micro-organisms in the soil. To the right are shown subsidiary cycles which animals and insectivorous plants are involved. Still further to the right are shown processes not belonging to the 'cycle' proper which lead to the introduction of additional nitrogen compounds from the nitrogen of the air; also those leading to loss of nitrogen compounds from the 'cycle' by liberation of nitrogen gas. The parts played by symbiotic micro-organisms in nitrogen exchanges are indicated below the main diagram.
There remains for consideration the most important direct source of nitrogenous food for green plants; namely, the small and fluctuating amounts present in the soil as nitrates or compounds of ammonium, both readily soluble in water, and hence present in the water in contact with the soil particles. The manner in which these inorganic compounds of nitrogen come to be present in all fertile soils is a single chapter in the fascinating story of that remarkable series of natural events usually described as the 'nitrogen cycle'.
The 'nitrogen cycle' is a chain of many links, each the life history of an organism or group of organisms living side by side with others, all differing sharply in their food requirements and the manner in which the raw materials of their food are obtained and utilized. Only in brief outline can this story be told here in order to make clear the respective parts played in the whole chain of events by green plants on the one hand and by certain non-green plants -- members of the soil microflora -- on the other. Let us begin with the nitrogen problem as it affects the diet sheet of the ordinary plant growing in soil.
The green plant is, in general, a 'nitrate organism', that is to say, it obtains the nitrogen required for nutrition and growth in mineral form as nitrates dissolved in the soil water. Most green plants can use also soluble salts of ammonium if these are available. Being extremely soluble, nitrates are quickly leached from the soil by rain. Ammonium salts are also readily soluble but less easily removed by leaching; since they are converted into nitrates by bacterial action during the nitrification process constantly taking place in fertile soils, their retention is only temporary. Since also both nitrates and salts of ammonium are in demand not only by green plants but by fungi and other members of the soil population, there is intense competition for these forms of available nitrogen in the soil and the supplies are often depleted more rapidly than they can be replaced by natural means. It is for this reason that the ordinary green plant growing in soil is confronted with a nitrogen problem, and for this reason also that the deficiency likely to be present in the case of crop plants must be made good by supplies of suitable manure.
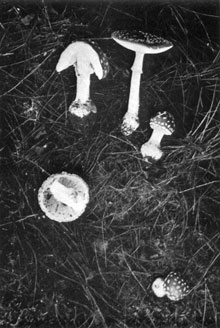
Plate 3. Sporophores of the Fly Agaric (Amanita muscaria) of various ages showing external features. Note the volva at the base of the stem and the ring, both of which are characteristic features of the genus Amanita.
|
Other chapters in the story of the nitrogen cycle tell how the nitrates absorbed by green plants are built up into plant proteins; how these, consumed by animals, become incorporated into those of the animal body, and how both plant and animal proteins after death as well as the nitrogen-containing excretions of the latter; during life, eventually accumulating in the soil, are converted again to ammonium salts and nitrates in course of their use as sources. of. food and energy by fungi, bacteria, and other members of the soil population.
In most soils and especially in fertile cultivated soils, the completion of the nitrogen cycle by the continued breakdown of some of the nitrogenous organic materials present in the soil humus leads in part to the formation of ammonia, a volatile substance some of which escapes into the atmosphere -- to be subsequently washed down in rain -- the remainder going into chemical combination to form ammonium salts. The last links in the chain lead to the conversion of these ammonium salts to nitrates once more and thus to completion of the nitrogen cycle.
The conversion of ammonium salts to nitrates takes place in two stages and is a characteristic of all fertile soils. The process is called nitrification and depends upon the vital activities and rather unusual food preferences of two closely associated kinds of bacteria, one of which, Nitrosomonas, consumes compounds of ammonia producing nitrites as an end-product, the other, Nitrobacter, using nitrites and incidentally converting them into nitrates. Whereas the ordinary green plant and the animal obtain the energy they require for vital activities by the slow combustion -- oxidation -- of carbon compounds, so giving rise to water and carbon dioxide gas as products of the complete respiration process, the bacteria responsible for this last link in the chain use compounds of nitrogen for the same purpose, oxidizing the ammonium salts to nitrates in two stages. The ordinary green plant is almost entirely dependent for the raw material of its nitrogenous food upon these remarkable activities.
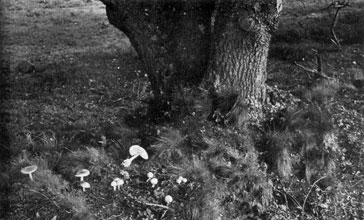
Plate 4. Young sporophores of Amanita phalloides, the Death Cap Fungus, near the base of an Oak tree. About one-tenth the natural size.
|
During this complicated cycle of events -- the conversion of nitrates into proteins by the green plant and of these subsequently back again to ammonium salts and nitrates by micro-organisms -- there is some loss of nitrogen owing to the activity of a few species of bacteria and other organisms that attack organic materials containing nitrogen or nitrates, breaking these down in either case in such a manner that some of the contained nitrogen is set free and returns to the air as nitrogen gas. This escape of nitrogen from chemical combination by denitrification as it is called, is compensated by the reverse process described as fixation of nitrogen likewise dependent on the life activities of a group of organisms -- certain highly specialized bacteria, probably some species of fungi; and a few of the simpler green plants or algae that can utilize nitrogen gas as a source of food and build it up into the proteins of their bodies. Some of the nitrogen-fixing bacteria, species of Azotobacter and Clostridium, live independently in the soil, others are responsible for the formation of nodules on roots, more rarely on leaves, and carry on their activities within the tissues of a host plant. Wherever it happens, the nitrogen thus brought into combination and built up into body materials eventually reaches the soil in the form of the organic compounds of which these are composed. In the diagram that illustrates the course of events in the nitrogen cycle, this direct loss and gain of nitrogen is shown as side chains passing to and from those that represent the main cycle.
And so the nitrogen cycle in nature comes full circle. The inorganic nitrogen of the air, a gas singularly inert in its relations with other substances, becomes an essential part of the fabric composing the bodies of plants and animals, in particular of those complex substances, the proteins, that form the chemical basis of all living matter. It may remain thus caught within the nitrogen cycle proper, passing from protein to nitrate and back again to protein through those phases of vital and chemical activity here briefly described, now generally known to science as ammonification, nitrification, and protein synthesis; or it may return once more to the air as free nitrogen gas until again brought into circulation through the process known as nitrogen-fixation (see diagram).
As well as carbon, nitrogen, and the elements present in water, the plant has certain mineral food requirements. For all green plants, phosphorus, potassium, sulphur, calcium, magnesium, and iron are essential; in addition, many species have been found to require minute quantities of other and rarer elements, boron, zinc, manganese, even traces of copper. Because of the small quantities required, these latter are often now called 'trace elements' when considered in relation to plant nutrition, the need for them varying in kind and degree in different species of plants and on different soils. All these mineral nutrients must be present in forms soluble in water or otherwise available for root absorption. Some of them, e.g. the metallic elements, calcium, magnesium, and iron, are usually present in abundance in most soils in the form of compounds such as sulphates, phosphates, or nitrates, although rare cases are known in which even the available magnesium present is insufficient for crop requirements. Others, notably phosphorus and the 'trace elements' are, like nitrogen, often deficient in amount and must be supplied artificially, either directly in suitable forms, or as humus or organic materials of one kind or another from which they will be released eventually as a result of chemical changes brought about by the bacteria, fungi, and other members of the soil population that attack organic residues.
The vital activities of the many lower organisms responsible for breaking down of complex organic materials into simpler chemical substances is described as decay when carried on in well-aerated conditions, and as putrefaction when aeration is defective, different sets of organisms being concerned in the two cases with different end-products resulting from their activities.
Under normal, well-aerated conditions, the soil humus is thus formed, a friable dark-coloured mixture of organic materials, complex in origin, complicated and inconstant in chemical constitution.
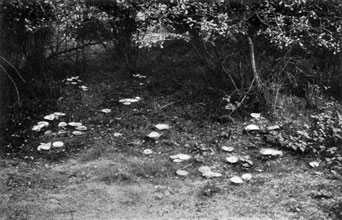
Plate 5. Sporophores of a species of Lactarius on the
ground beneath beech and willow.
|
To summarize the gist of all this. Almost alone among living organisms, the green plant can utilize as a direct source of carbon the constantly renewed supply present in the atmosphere as carbon-dioxide gas.
For sources of nitrogen, green plants are, in general, restricted to combined nitrogen in the form of nitrates or salts of ammonium in the soil, the former derived from the combination of nitric acid with various mineral bases in soil such as lime or soda, the latter formed by combination of ammonia with various acids.
Renewal of the supply of nitrate in soils depends upon a series of intricate processes, each involving the vital and often highly specialized activities of living organisms. Moreover, nitrates and ammonium salts are in demand also by members of the soil microflora, and being soluble, are liable to be washed out of the soil by rain.
For all these reasons, the supply of compounds of nitrogen in the soil available for green plants tends to fall below their full capacity of utilization, and may become so deficient in the case of crops that it is necessary to supplement it artificially, the same being true of certain mineral nutrients, notably, suitable compounds of phosphorus and potassium. This brings our minds back to the importance of humus as a source of plant food.
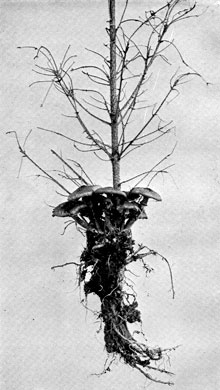
Plate 6. The Honey Agaric, Armillaria mellea. Group of sporophores growing at the base of a small spruce tree killed by the mycelium of the fungus. About one-sixth natural size.
|
The soil humus is a mixture of organic materials, complex in chemical constitution and continually undergoing changes in respect to this. Over and above the part played in modifying the water-holding, water-yielding, and other physical properties of the soil, humus is, therefore, a great reservoir of organic carbon and nitrogen compounds, with which are bound up chemically the various mineral elements also required by plants.
As well as the roots of plants forming the surface vegetation, the soil contains a teeming population of animals and plants. Of these, the minute forms that constitute the microfauna and microflora have their home mainly in the soil humus and carry on within it their manifold vital activities. On some of the latter depend the conversion of the raw organic debris into humus in the first instance; on others the gradual breaking down and constant changes in the humus constituents, whereby are set free nitrogenous materials of simpler chemical composition that play their part in various phases of the nitrogen cycle, liberating at the same time the mineral components originally present.
Among these soil activities, those carried on by the mycelium of the toadstool-forming fungi must play an important part. It may be noted that these fungi, in common with other members of the soil population, are competing with roots of the higher plants for water and certain nutrients. Unlike the latter, however, they can find the carbon they require in sugar and other organic substances formed in the soil during the decomposition of plant and animal residues; their nitrogen also in various organic materials of similar origin before these reach the final stages of ammonium salts and nitrates, although the latter are also utilized. They are therefore competing with the. roots of green plants for water and mineral salts and to some extent also for nitrates and salts of ammonium. Fungi have an advantage over green plants in respect to access to sources of carbon, nitrogen, and mineral salts, but depend absolutely upon their green competitors for the provision of supplies of carbon compounds, because all the carbon contained In organic compounds in the soil or elsewhere is derived ultimately from the carbon-dioxide of the air brought into organic circulation by the photo-synthetic activity of green plants. A complicated and delicately adjusted system indeed, and from the nature of the case, one easily altered or thrown out of gear by changes affecting the character of the soil or the composition of the vegetation living upon and within it.
Next chapter
Back to the Small Farms Library Index
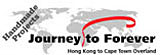
Community development | Rural development
City farms | Organic gardening | Composting | Small farms | Biofuel | Solar box cookers
Trees, soil and water | Seeds of the world | Appropriate technology | Project vehicles
Home | What people are saying about us | About Handmade Projects
Projects | Internet | Schools projects | Sitemap | Site Search | Donations | Contact us
|